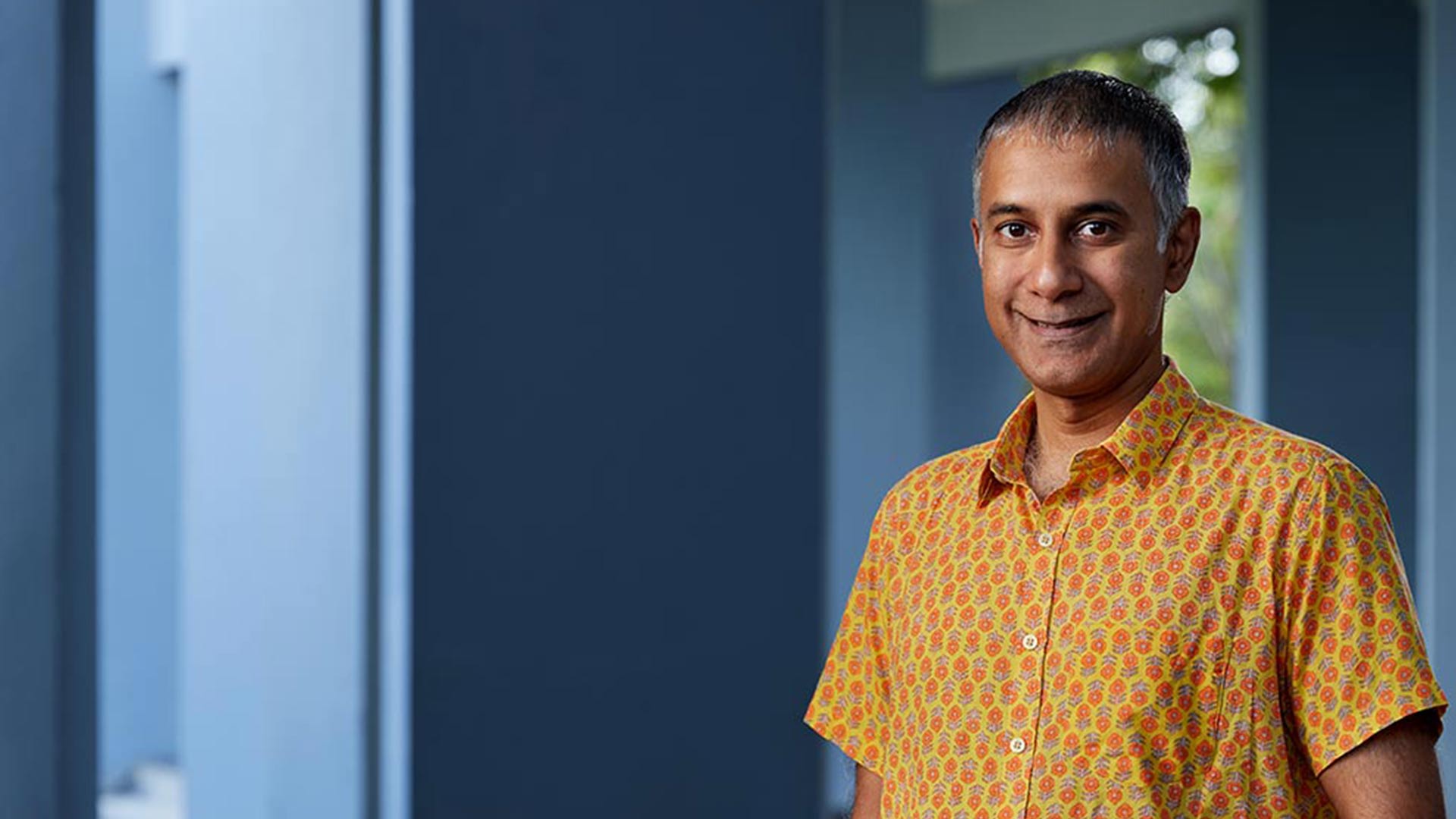
In conversation with Mukund Thattai
Netra Prakash
September 20, 2024
Prof. Mukund Thattai won the Infosys Prize in Physical Sciences in 2023 for his research on evolutionary cell biology. In this exclusive interview, Prof. Thattai discusses everything from his work in this fascinating field of science, to the importance of interdisciplinary research, to his advice for high schoolers aspiring to be scientists.
1. You won the Infosys Prize for your work in the field of evolutionary biology. Can you explain the latest developments in this field in layperson’s terms?
Evolutionary biology is a mature and complex field, dealing with several interlocking ideas. When Darwin came to understand the laws and processes of evolution, he was looking at large organisms: plants and animals. But of course, evolution works all the way down to smaller scales of life. We know now that even viruses, bacteria, and single-celled eukaryotes undergo evolution. At least for a hundred years, in fact, we’ve known the mechanism of evolution from the cellular perspective. We’ve come to understand how mutations arise, how genes are passed down from parents to offspring, how selection works, and therefore how the traits of organisms change over time to make them better suited to their environments.
We are now trying to understand how the organisation of the cell itself arose in the deep past. How did the eukaryote come into being two billion years ago? In the same way that we reconstructed the history of humans by studying human fossils and retracing the origin of apes and so on, we’re trying to reconstruct the history of the eukaryotic cell. The reason this is more difficult is because unlike humans or dinosaurs, cells very rarely leave fossils.
Furthermore, human history is rather recent, while cellular history goes back billions of years. You have to use a different approach to understand the evolution of cells.
One approach is to use the genome. All organisms carry genomes, and in some sense these are all descended from some ancestral states through small, accumulated changes. If you look at a large enough variety of genomes today, you can retrace them to see what the ancestral states were in the past.
This domain of evolutionary biology has taken off in recent times due to the increased availability of genomes: the process of sequencing genomes has become more reliable and cheap. Also, people are rapidly discovering new kinds of organisms that we didn’t know about before. And these aren’t just close variations of existing groups, but entirely new species! Adding a new branch to a tree means you can push the tree back further in the past, to see more ancient details.
2. You’ve previously mentioned your interest in curiosity, rather than application, driven research. Could you please elaborate on the value of such research?
The way I see it, science has two pieces to it. One is the process of discovery, wherein people go after questions that interest them — questions that are often rather open-ended. Questions about the movements of stars and planets, for example, are very abstract, and yet they’ve captivated humans ever since we were humans.
But science is equally a powerful way to develop technology. Technology, of course, has enormous implications for how we organise ourselves as individuals, as societies, and as a civilisation. And technology has the power to improve our lives.
We don’t necessarily do science in order to develop technology, but there are pieces of science that have led to incredibly useful technological applications: electricity, jet planes, satellites, and protein-based drugs, to name a handful. All these developments were initially based on a scientific idea for which people never even conceived that these would be the applications. CRISPR, which is used now to modify genomes, was initially discovered through a curiosity-based study on how bacteria defend themselves against infection. No connection to how we use it today!
So I’m not saying applications aren’t important, but that applications cannot be foreseen. Focusing exclusively on applications means that you cut off the original source of ideas: curiosity-driven science. For example, if everybody’s convinced that they want to cure cancer, and that every single biologist on earth must work only towards that, I guarantee that they miss out on discoveries which could prove important for finding a cure.
3. You have used physics and computer science to study cellular biology. Would you agree, therefore, that seemingly different branches of science are often interdependent?
Absolutely. The process of doing science itself is common across all sciences. You have ideas, you test them through experiments, and you develop mathematical models to state your hypotheses in more rigorous terms. Those steps are common whether you’re studying biology, chemistry, or physics. It’s only the set of basics you need to know in each field which differs.
I study things at the level of a cell. Obviously, inside a cell there are molecules, and molecules interact with each other, and those interactions are governed by the laws of physics. So to understand a cell at a deep level, you have to account for the physics within it as well. A biologist may be interested in the benefits of a cell’s functions, whereas a physicist may be more interested in the molecular mechanisms behind those functions. But it’s a spectrum. There’s no hard line between these sciences.
4. You yourself first studied physics at undergraduate, masters, and doctorate levels, before you joined NCBS. Can you share a bit about your unconventional path to working in biology?
You’re right. I did my PhD in MIT’s physics department, where I’d initially gone to study condensed matter physics: the physics of large collections of atoms and molecules and their interactions. It turns out that one of the places where these interactions occur is in cells. In many ways, these interactions are much more interesting within cells than in generic, non-living matter.
The specific question I tackled was of stochastic gene expression. When a cell divides into two daughter cells, they are nearly identical, and yet they end up functioning differently. What causes this difference?
The randomness intrinsic to the creation and destruction of cells is completely under the purview of physics. So as a physicist, I started modelling this process. We built a little biology lab in the physics departement, set up microscopes, and grew cells and modified their genes, essentially learning biology along the way. What really motivated me was that the models I made actually predicted what the experiments later did. With that as my launchpad, I had a great introduction to biology, and joined NCBS after finishing my PhD.
There was no one moment where I gave up physics to pursue biology. I still do physics, but I now apply what I know from physics to biological systems.
5. Was it easy, in your experience, to transition from one field to another?
It wasn’t easy to go from physics to biology. I’d never learnt biology beyond tenth grade in school, so I had to teach myself the subject from scratch. I spoke to people, read books, and attended lectures. Getting into biology from physics is exciting and enriching, but it requires patience, time, and humility to understand the language of a whole new field.
When you’re working in the core of a very mature field of physics, there is a roadmap. You follow the path of those before you. You study certain books in a certain order, and then start your work in a certain way. It is hard, yes, but there’s a roadmap.
There’s no clear path to working in an interdisciplinary area. Everyone gets to it in their own way: a lot of accident, a lot of serendipity. But it’s enjoyable, and in my opinion, leads to much more scientific progress overall. Once the mature core of a field is saturated, new discoveries are surely at the periphery. So these interdisciplinary areas become fertile ground to find questions people haven’t even asked before, let alone answered.
6. What advice would you like to give high schoolers aspiring to work in science as they forge their own career paths?
My first, simple piece of advice: you’ve got to learn maths. You can’t give it up. It’s the language of science, no matter what science you do. Don’t let anybody tell you you don’t need maths for biology!
Also, keep your options open for as long as possible. What you learn in school may not accurately represent a field. When you decide you don’t like physics, sure, you may not enjoy it in school. But had it been taught differently, you might have felt differently. Don’t make up your mind about what you like and dislike too early.
Conversely, if you think you have an aptitude for something, chase it at the highest level. If you’re skilled at maths, nothing prevents you from going beyond the curriculum. Pick up some books, find a mentor, start a project, and really explore beyond the classroom. The more time you spend with a field of study, the easier it is to gauge whether it’s really for you.
People often ask me for advice on what their kids should study in college. But that’s not my place to say. Instead, I’d turn it around, and ask you to look into yourself. What do you think is your strongest skill? What is your deepest interest? And what excites you the most?