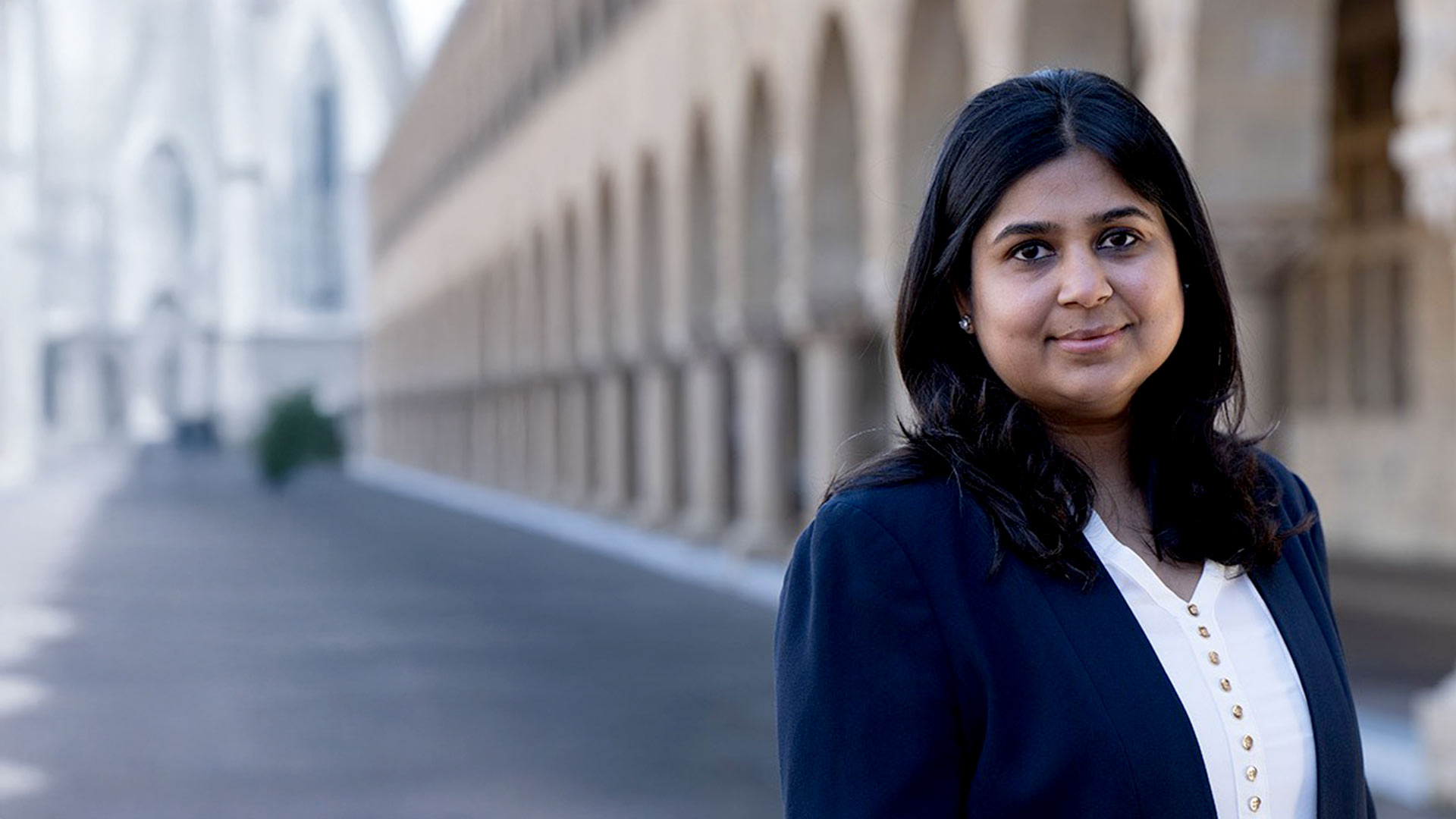
In conversation with Vedika Khemani
Netra Prakash
02 February, 2025
Prof. Vedika Khemani won the Infosys Prize 2024 in Physical Sciences for her groundbreaking work in non-equilibrium many-body physics, specifically the discovery of time crystals. In this interview, Prof. Khemani explains her work to a high school audience and shares her advice for those aspiring to succeed in science.
1. You won the Infosys Prize in Physical Sciences for your work in non-equilibrium many-body physics. Can you explain this field to a high schooler?
The focal point of many-body or condensed matter physics is understanding the emergent properties of systems with many degrees of freedom, many particles, many entities.
Each entity individually may be simple, but from the interactions among many, a complex system emerges. Think of an ant colony, a flock of birds, the human brain, even human society itself. If you study a single ant, a single bird, a single neuron, or a single individual, you can’t predict what collective phenomena arising from their interactions will look like. Emergence, therefore, is the idea of complexity arising from simplicity.
Traditionally, we use many-body systems to study phases of matter. A single water molecule is neither solid, nor liquid, nor gas. It takes many billions of water molecules coming together at a certain temperature and pressure to determine the phase of matter. Many-body physics has now evolved from studying conventional solids, liquids, and gases, to much more exotic phases like superconductors, semiconductors, liquid crystals — basically quantum materials.
But when you want to make a statement about a large number of particles, you can’t individually track each particle, so you rely on the framework of equilibrium statistical mechanics. Instead of analysing individual particles, you track the coarse-grain macroscopic properties of the system in equilibrium. However, equilibrium phases only comprise a tiny fraction of all possible states of a quantum system. If you look at the world around you, everything’s always changing. Nothing’s really in equilibrium. If you open a textbook on quantum mechanics, the most general description of a quantum mechanical system is an out-of-equilibrium process. It’s either time-evolving, according to Shrodinger’s equation, or it’s being subject to measurements, which themselves cause changes in its state. So we wonder, why are we limited to studying phases of quantum materials in equilibrium? What do emergent phenomena look like out of equilibrium?
These questions are the essence of non-equilibrium many-body physics.
2. What led you to explore this particular branch of physics?
Why are we asking the question of non-equilibrium many-body physics now? If the textbook description of Schrodinger’s equation includes time evolution and measurements, then why has all of many-body physics so far focused on systems in equilibrium? It’s because traditionally, that’s the regime which is easiest for an experimentalist to probe. You start with some material in equilibrium, you tickle it a little bit, and you see how the system responds to that weak perturbation away from equilibrium — but you’re always working around equilibrium.
But over the last ten years or so, there’s been tremendous progress motivated by the quest to build quantum computers. Developments towards this goal have already led to a new class of quantum experiments, with systems of many quantum particles. Instead of particles just hanging out in a solid, behaving in accordance with nature, an experimentalist can now control how they interact. You can literally grab an individual atom, get it to talk to some other atom in some prescribed way, then get these atoms to talk to another group, and another group, and so on. We’re still looking at many-body emergent phenomena, but we now have control over individual entities.
There are fundamental questions in quantum dynamics, in many-body physics, which have been left unanswered. And thanks to the recent focus on these new experimental platforms, we can finally probe these questions through experiment.
While I was doing my PhD, this field was getting to an exciting place. It was brand new and there was not much literature to absorb before going forward. The experiments were just getting going. It felt like a great opportunity to start asking fundamental theoretical questions, now grounded in new experiments.
3. You are known for your collaborative research style. According to you, what role does teamwork play in scientific research?
I think it plays a really important role. Working with motivated people of all career stages is what makes science fun for me.
The most interesting work can come from cross-pollination between different ways of thinking. If there’s an area where I have expertise, and I suspect there’s some insight that can come from combining this area with a parallel stream of work, then I collaborate with an expert in that stream. We then ask a question that neither alone could have asked, so both parties learn a lot from the process.
Another kind of collaboration is working with experimentalists. Even though I’m a theoretical physicist, I work with different experimental groups to bring theoretical ideas to reality. Things that seem easy in theory end up being difficult in a real physical system, and things that I think are difficult end up being easy. Understanding what an experiment can do helps sharpen my theory and generate new questions.
4. You spoke about exploring new topics, so what is next for you?
I actually put out a paper just last month which brought several streams together!
Error correction is an essential part of quantum computing. Quantum bits are extremely fragile, and a quantum computer works only if it can sustain their delicate entangled state. But anytime an environmental agent pokes at a quantum system, the entanglement collapses. That’s why there’s a whole field called error correction, which tries to encode the quantum state in a way that is robust to all these environmental influences. This is an area where there’s been a lot of cross-talk between many-body physics and quantum information.
Recently, people have formulated brand new models of quantum error correction, which are fantastic error correcting codes. Interestingly enough, these codes live on weird non-Euclidean geometries such as expander graphs. We may live in three spatial dimensions, but when you can get your atoms to interact however you like by design, then you’re no longer limited to these dimensions — you can explore completely general graphs and networks.
I got intrigued, and wondered, what’s the physics behind these good codes? We found that these models realise a brand new phase of matter called a topological quantum spin glass.
This project has been really fun for me, because we started off with a development in quantum error correction in computer science, which led us to the maths of expander graphs, which in turn led us to the statistical mechanics of spin glasses and neural networks. All of these different fields came together, and I think there’s so much more to learn here.
5. Finally, what advice would you give to high schoolers aspiring to be successful scientists themselves?
Get obsessed. Within every scientific problem, if you look deep enough, there’s some amazing insight to be found. So find a problem where you enjoy what it takes to go deeper, and fixate on it. Don’t settle for a superficial explanation, don’t sit back and say, “yes, this is good enough.” When you really strive for excellence, I’m sure you’ll find something meaningful.